
2002) and nutrient transporters ( Buddington & Diamond, 1989 Ferraris & Diamond, 1989 Diamond & Hammond, 1992, 1994 Diamond, 1993 Hammond et al. My colleagues and I have measured safety factors of intestinal brush-border hydrolases ( Weiss et al. 1996, 1997 Staples & Suarez, 1997) have published insightful studies of animals operating with various metabolic rates and corresponding variation in safety factors, and have also solved the paradox of apparently exorbitantly high safety factors of biochemical reactions operating near equilibrium. Suarez and colleagues ( Suarez, 1992, 1996, 1998 Suarez et al.

DONT STARVE OPTIMAL LAYOUT SERIES
1987, 1991, 1998 Weibel, 2000), whose measurements of the key physiological and anatomical parameters in mammalian systems for aerobic exercise are unique in their comprehensive scope, and who introduced the term ‘symmorphosis’ to describe series systems with safety factors close to 1.0 (see below). 1984), who applied the engineering concept of safety factors to biological systems, and who developed an optimality model for systems consisting of series components and the long series of experimental papers by Taylor, Weibel and colleagues (e.g. The two pioneering sets of studies that founded this field have been the theoretical papers by Alexander (1981, 1984, 1997 Alexander et al. On the other hand, physiologists are in a position to measure the evolutionary costs that evolutionary biologists routinely postulate but have rarely have been able to measure. On the one hand, the concepts of ‘ultimate causation’ and ‘evolutionary design’, which I shall discuss below, have been exploited routinely in evolutionary biology but rarely in physiology. By this approach, physiologists and evolutionary biologists may be able to contribute solutions to each others’ problems. It uses evolutionary reasoning to understand, in terms of ultimate rather than proximate causation, why any particular physiological or anatomical quantity has the numerical value that it does, rather than some higher or lower value. Those costs are likely to involve wasted energy or space for large or expensive components, but opportunity costs of wasted space at the molecular level for minor components.Ī research program that has been emerging at the interface between physiology and evolutionary biology is the approach termed quantitative evolutionary design. The modest sizes of safety factors imply the existence of costs that penalize excess capacities. Unsolved questions include safety factors of series systems, parallel or branched pathways, elements with multiple functions, enzyme reaction chains, and equilibrium enzymes.
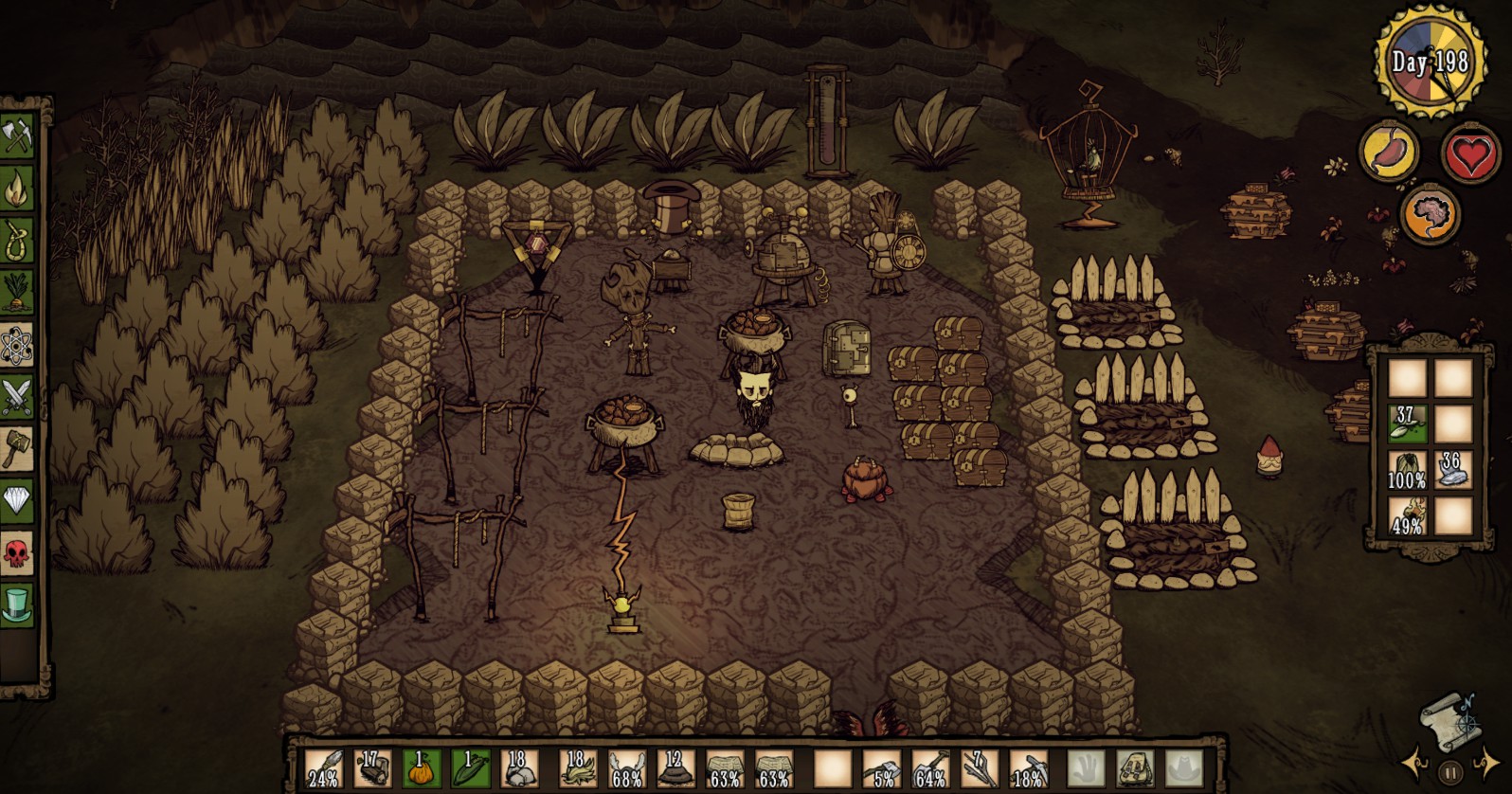


Adaptive regulation of many biological systems involves capacity increases with increasing load several quantitative examples suggest sublinear increases, such that safety factors decrease towards 1.0. Safety factors increase with coefficients of variation of load and capacity, with capacity deterioration with time, and with cost of failure, and decrease with costs of initial construction, maintenance, operation, and opportunity. Safety factors serve to minimize the overlap zone (resulting in performance failure) between the low tail of capacity distributions and the high tail of load distributions. Familiar examples of engineered safety factors include those of buildings, bridges and elevators (lifts), while biological examples include factors of bones and other structural elements, of enzymes and transporters, and of organ metabolic performances. Ratios of capacities to loads, defined as safety factors, fall in the range 1.2-10 for most engineered and biological components, even though engineered safety factors are specified intentionally by humans while biological safety factors arise through natural selection. excesses of capacities over natural loads. The field of quantitative evolutionary design uses evolutionary reasoning (in terms of natural selection and ultimate causation) to understand the magnitudes of biological reserve capacities, i.e.
